CESR Seed Grants
CESR awards support for 18-month collaborative research projects in sustainability approximately annually. Funding levels range from $65 to 80k. Supported by funding from Leslie and Mac McQuown, the CESR Seed Funding program fosters innovative, high-risk research that fosters new faculty collaborations, and provides support for graduate student(s) and/or postdoctoral fellow(s). Results from these projects aim to provide preliminary data to support proposals for funding from federal agencies, foundations, and/or industry. Funded teams present their results annually at a spring poster session. Applications for the Seed Grant program are currently closed.
2023 Awarded Teams
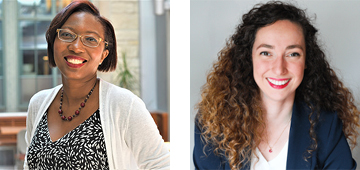
Co-Lead PI: Ludmilla Aristilde, Associate Professor of Civil and Environmental Engineering, Chemical and Biological Engineering (by courtesy), and Earth and Planetary Sciences (by courtesy)
Co-Lead PI: Cécile Chazot, Assistant Professor of Materials Science and Engineering and Julia Weertman Professor in Materials Science and Engineering
The production, consumption, and disposal of single-use plastics pose several problems for the environment, including the persistence of plastic debris in the ocean. In recent years, the biological production of polyesters has emerged as environmentally friendly alternatives to petroleum-derived polymers in plastics packaging, due to their biodegradability and tailorable mechanical properties. However, the thermal stability of these bio-derived polyesters is too low for thermal recycling, thus remaining as single-use plastics. To circumvent this issue, we will explore the grafting of the bacteria-produced polyesters onto the thermally stable biopolymer chitosan, as a unique approach to circular production of thermally processable and recyclable bio-copolymers with increased degradation temperature. We aim to develop a new class of recyclable, thermally processable, and biodegradable copolymers, called thermoplastic bio-copolymers for packaging applications as part of the circular economy portfolio essential to a sustainable future.
Matthew Grayson and Bin Chen
Introducing AC Photo-Hall Method: Separating Electron/Hole Mobilities in Perovskite Photovoltaics
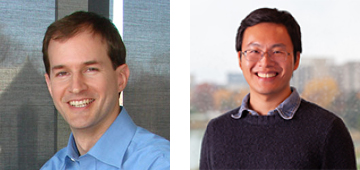
Lead PI: Matthew Grayson, Professor of Electrical and Computer Engineering
Co-PI: Bin Chen, Research Assistant Professor of Chemistry
Perovskite photovoltaics have sparked a resurgence in solar cell research: they are cheap, easy to manufacture, and highly efficient, but given their relative novelty, we don’t yet know how to measure certain important properties to improve them. This research will develop a new research method to measure how fast the charges can move in perovskites. Because solar cells convert light energy into freely moving positive and negative charges, the faster those charges can move, the more efficient the solar cell. But the state-of-the-art method for separately measuring the mobilities of the positive and negative charges, the photo-Hall effect, is challenging, potentially yielding inaccurate results and preventing a proper understanding of how to improve these materials. Here, a new experimental technique is proposed that uses an oscillating light source to modulate the photo-Hall signal, thereby significantly improving the accuracy of the measurement. We will use high-quality perovskite single-crystal and thin film samples from co-PI Chen that achieved world-record efficiency solar devices. We will then experimentally verify the mobilities obtained by AC photo-Hall with mobility spectral analysis, a complementary technique developed by PI Grayson. The successful completion of this project will verify the world’s first accurate characterization of electron and hole mobilities in lead-halide perovskites using this newly introduced method.
Keith Tyo and Ted Sargent
Sustainable Chemical Production by Coupling Electrochemistry and Synthetic Biology
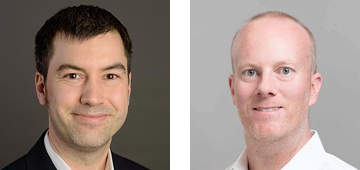
Lead PI: Keith Tyo, Associate Professor of Chemical and Biological Engineering
Co-PI: Ted Sargent, Lynn Hopton Davis and Greg Davis Professor of Chemistry, Professor of Electrical and Computer Engineering, and Co-Executive Director of the Paula M. Trienens Institute for Sustainability and Energy
Current production of fuels and chemicals that are needed by society contributes to greenhouse gas release and is not sustainable. Our vision is to find a new, sustainable way to use carbon dioxide (a greenhouse gas) and clean electricity to make these essentials, including oils, chemicals, and plastics. We will combine two methods: one that uses electricity to convert carbon dioxide into a building block called acetate, and another that uses bacteria to turn the acetate into more complicated chemicals. The problem is the process used to make acetate from carbon dioxide is done in harsh conditions that would kill the bacteria. The first step, then, is to find the right balance for both methods to work well together. We will use the demonstration funded by this seed grant to apply for large federal grants where we can further optimize the electrochemistry while also engineering the bacteria to make oil, and eventually other chemicals.
2022 Awarded Teams
Scott Barnett and Linsey Seitz
Application of Exsolution Electrodes to Low-Temperature Electrochemical Cells for Hydrogen Production and Utilization
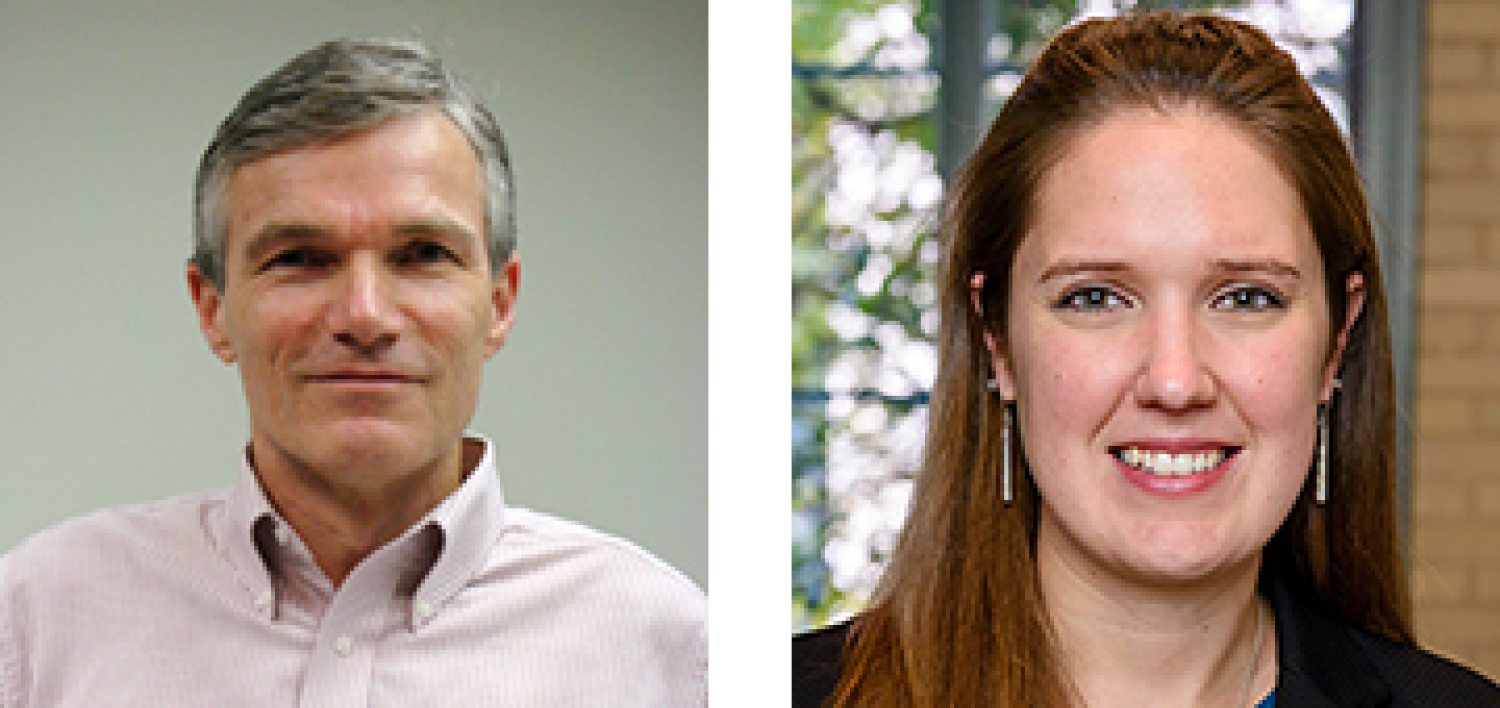
Lead PI: Scott Barnett, Professor, Department of Materials Science and Engineering
Co-PI: Linsey Seitz, Assistant Professor, Department of Chemical & Biological Engineering
As we address the imminent threats of global climate change and work to transition from largely fossil fuel-based energy systems to renewable electricity-based energy systems, it is imperative to develop technologies for efficient conversion and storage of these intermittent energy sources. However, as these emerging technologies are scaled to meet our massive energy demand, they will drive increasing pressure on various materials that are required to support them. Therefore, to enable a truly sustainable and revolutionary energy transition, we must work to eliminate or reduce the precious metal and critical mineral content of these technology components, while preserving or enhancing their performance. In particular, hydrogen generation or utilization using low-temperature water electrolysis or fuel cells typically requires relatively large amounts of scarce precious metals. Our work will pursue the first ever application of a new class of electrode material, previously developed for high-temperature electrochemical cells, in low-temperature cells. We aim to explore whether the promising properties and performance of these materials as demonstrated in high-temperature environments, will effectively translate and enhance low-temperature processes for green hydrogen production and utilization, even while reducing the need for precious metals. This approach could mitigate the growing demand on materials sourcing that is caused by scaling up of sustainable hydrogen technologies.
Neal Blair and George Wells
Agricultural tile drains as a massive, uncharacterized new environment for microbially-driven C-cycling
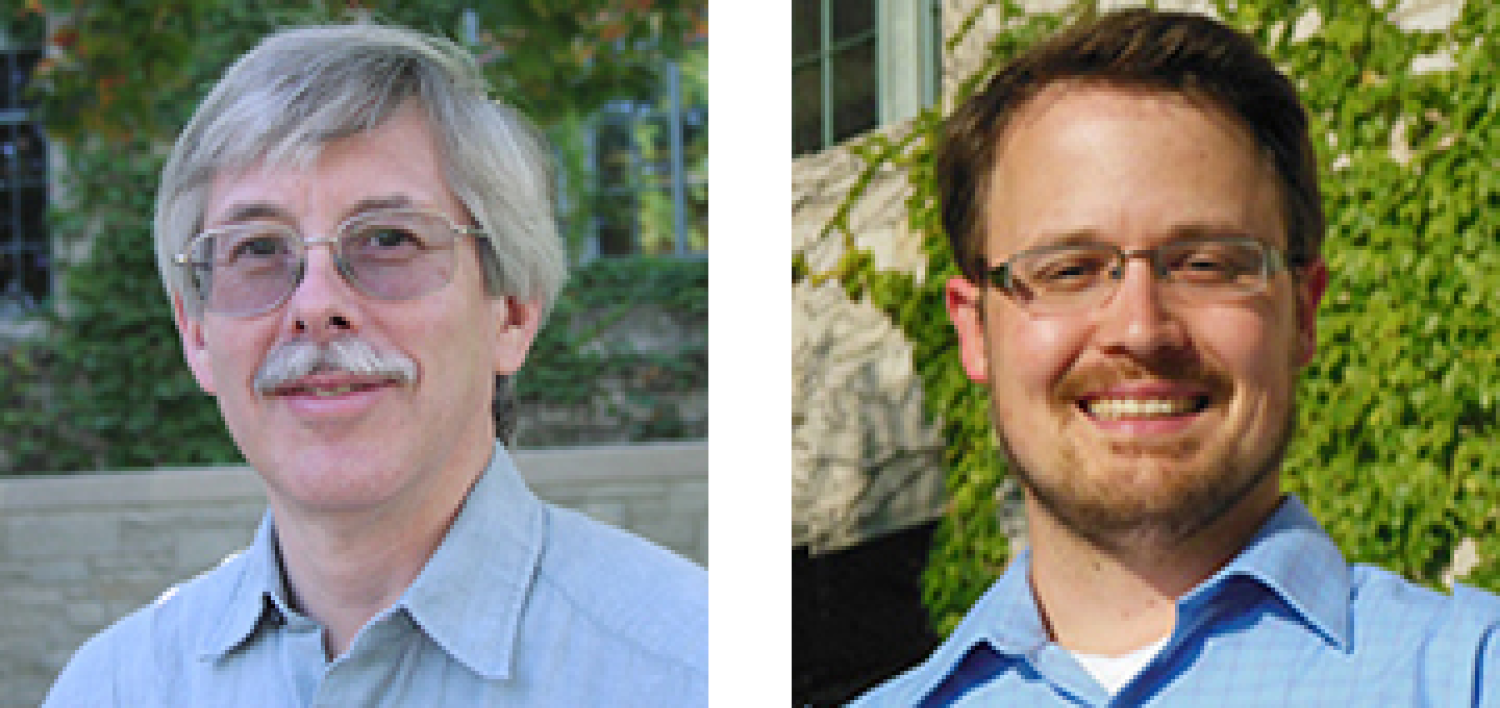
Lead PI: Neil Blair, Professor, Departments of Earth and Planetary Sciences and of Civil and Environmental Engineering
Co-PI: George Wells, Associate Professor, Department of Civil and Environmental Engineering
Subsurface drainage systems (tile drains) are widely installed in flat, periodically wet agricultural areas to limit soil flooding. In the U.S. alone, approximately 56 million acres of farmland are drained, making this practice one of the largest hydrologic engineering endeavors by area in North America, and yet it is one of the most invisible. Tile drains contribute 40-95% of the water discharge in agricultural watersheds, and in doing so, export excess fertilizer downstream. The Dead Zone in the Gulf of Mexico is amplified by tile drainage. We hypothesize that the drainage system has created a massive uncharacterized underground ecosystem by transporting atmospheric O2 into the subsurface soils during dry periods. The influx of O2 into otherwise O2-poor soils may support novel microbial ecosystems that influence the C- and N-cycles. We plan to test our hypothesis with a combination of genomics and chemical studies to determine what bacteria are present and what they are doing. Because of the areal extent of tile drain ecosystem, it may prove to be an important contributor to global C and N budgets. The contribution will only increase in time as the need for more food and crop-derived biofuel production builds. Agricultural sustainability in the face of climate change may depend on tile drain systems and our knowledge of them. This project brings together two faculty, Neal Blair who specializes in C-cycle studies in the natural environment, and George Wells, an expert in the microbial processes of engineered systems. The project is an outgrowth of a proposal by Katie DuRussel who is an Environmental Engineering Ph.D. student and will be supported by the funding.
Sam Kriegman and Ryan Truby
Sustainable Design and Fabrication of Intelligent Robots
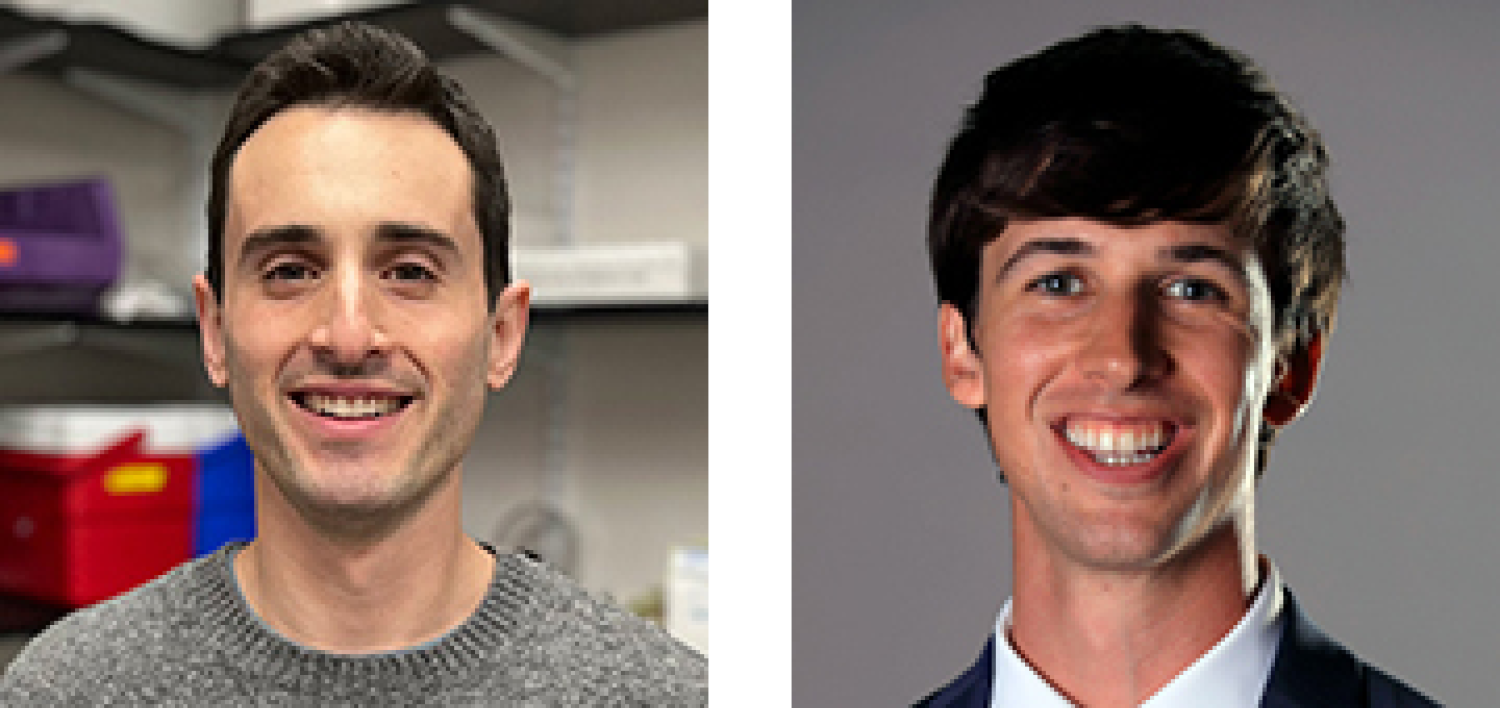
Co-Lead PI: Sam Kriegman, Assistant Professor, Departments of Computer Science, Mechanical Engineering, and of Chemical and Biological Engineering
Co-Lead PI: Ryan Truby, Assistant Professor, Departments of Materials Science & Engineering and of Mechanical Engineering
Although computational efficiency is improving for training robots, the design and fabrication of robots remains inefficient and unsustainable: Days of supercomputing—with carbon emissions equivalent to powering a home for several years in the United States—are required to achieve robots capable of the simplest behaviors, and considerable waste (plastics, toxic metals, harmful chemicals) must be discarded with each fabricated prototype. This is an unsustainable path for robotics that may never lead to truly intelligent machines. Support from CESR will allow us to reduce the computational, financial, environmental, and social costs of robot design, as well as improve robotics and AI technologies themselves. To do so, we will develop a computationally-efficient, low-waste pipeline for generating and printing novel sensorized robots. This will lay the groundwork for a sustainable path to intelligent robots, which may themselves be optimized to carry out the tasks necessary to sustain humanity and the biosphere.
Jeffrey Lopez and Dayne Swearer
Solvated Electrons for Direct Solution Process Recycling of Lithium-Ion Batteries
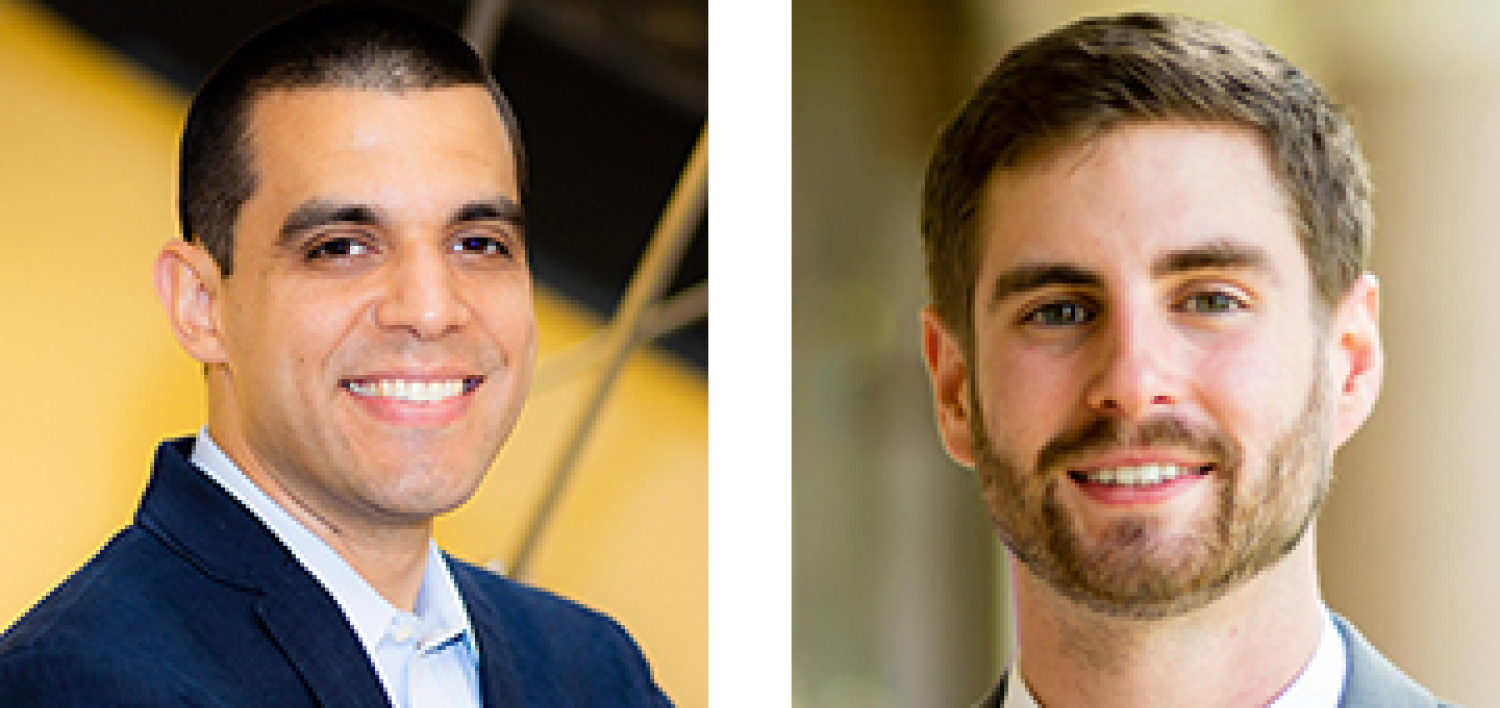
Lead PI: Jeffrey Lopez, Assistant Professor, Department of Chemical and Biological Engineering
Co-PI: Dayne Swearer, Assistant Professor, Departments of Chemisty and of Chemical and Biological Engineering
With the growing adoption of electric vehicles and the installation of grid scale energy storage, there is an impending influx of battery waste that will only continue to grow. To manage this waste and recover valuable materials from batteries at the end of their service life, battery recycling is of critical importance. Current recycling approaches break down the battery materials into their individual components so that new active materials can be synthesized and sent off for production. This approach is energy and resource intensive. Here we are working to leverage solvated electron solutions to efficiently recycle battery cathode materials directly in their existing particle form. Identifying direct recycling methods will be a critical step forward toward a circular lithium-ion battery economy.
David Morton and Ermin Wei
High-fidelity reliability assessment methods for low-carbon electric power systems
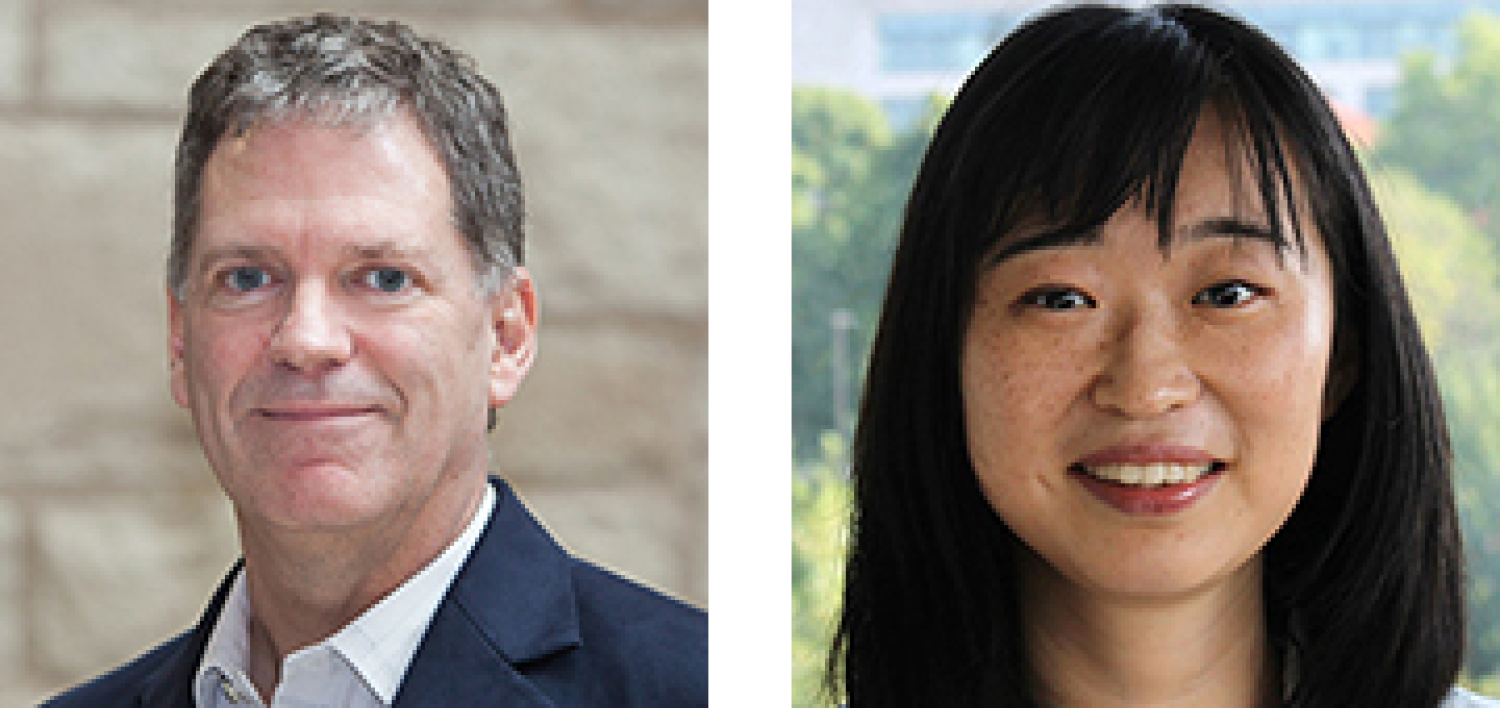
Lead PI: David Morton, David A. and Karen Richards Sachs Professor, Department of Industrial Engineering and Management Sciences
Co-PI: Ermin Wei, Assistant Professor, Departments of Electrical & Computer Engineering and of Industrial Engineering and Management Sciences
Combating climate change requires that fossil fuel-based electricity generation be replaced by increasing shares of wind power, solar power, energy storage, and flexible demand, whose operations differ from traditional power plants. Current methods for measuring system reliability were not developed with low-carbon grids in mind. Because reliability modeling helps to determine which, and how many, power plants are built, discrepancies between model assumptions and real-world operations can harm reliability and result in widespread power outages. High-fidelity modeling of grid operations is computationally challenging due to the need to simulate operations for tens of thousands of scenarios. To overcome this hurdle, we propose a computationally tractable, optimization-based scheme to automate identification of scenarios that can compromise system reliability. This is challenging because whether a scenario compromises system reliability depends on the system’s operational decisions. Meeting this challenge will allow us to run a realistic model of a grid’s operations on a much smaller set of scenarios, leading to a more accurate assessment of system reliability. Success with the proposed work is a step on the path to providing better investment incentives for transitioning to a reliable, low-carbon electric power system.
2021 Awarded Teams
Oluwaseyi Balogun and G Jeffrey Snyder
Towards Engineering Metamaterials for Sustainable Energy Solutions: Local Thermal Properties of Grain Boundaries in Polycrystalline Materials
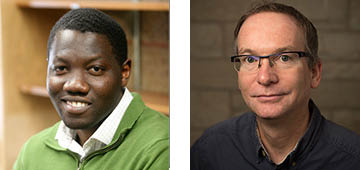
Lead-PI: Oluwaseyi Balogun, Associate Professor, Department of Civil and Environmental Engineering and Department of Mechanical Engineering
Co-PI: G Jeffrey Snyder, Professor, Department of Materials Science and Engineering
Grain boundaries (GBs) and interfaces are critical microstructural components that control the performance of electronic and energy materials. In particular, GBs are exploited in thermoelectric materials to promote the scattering of microscopic energy carriers like phonons, leading to reduced bulk thermal conductivity and enhanced energy harvesting performance. While reduced thermal conductivity in bulk thermoelectric materials has been widely reported, there is limited knowledge about the microscopic effects of GBs on heat flow, particularly at micro- and nanoscale distances on the order of the phonon mean free path (MFP). At these length scales, the impact of the GB structure, strain, and chemical composition on heat flow is expected to be significant. Using polycrystalline silicon as a testbed, the investigation team will (i) characterize the local thermal conductivity in the vicinity of individual GBs with a lateral spatial resolution of 10 nm; (ii) quantity the spatial extent of GBs influence on heat flow within each grain; and (iii) develop a modeling framework to facilitate rationale design of thermal metamaterials with tailored GBs for applications in heat management and thermoelectric energy harvesting. New insights on phonon-GB interaction gained in this project will impact the optimal design of thermal metamaterials with tailored nanostructures for broad applications, including sustainable thermoelectric energy harvesting.
Vinayak P. Dravid and Jean-François Gaillard
Towards Sustainable and Eco-Friendly Recovery of Critical Metals from Waste
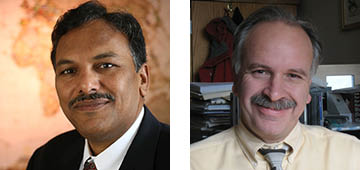
Lead-PI: Vinayak P. Dravid, Professor, Department of Materials Science and Engineering
Co-PI: Jean-François Gaillard, Professor, Department of Civil and Environmental Engineering
Metals are essential for powering the future of renewable energy in a sustainable manner. They are critical components for the development of essential technologies, such as solar panels, permanent magnets for wind turbines, batteries for electric vehicles, all of which are important pieces in minimizing the impact of industrialized society on climate. However, strategic metals for these new technologies are still mostly mined from the Earth at environmental and human costs. It is remarkable that a lot of industrial wastes that have been disposed/discarded in tailing and landfills contain significant concentrations of these metals and are not recovered. However, given the yearly exponential growth in mining some key metals, it is clear that sooner or later we will have to recycle 100% of the metals that we have extracted. Developing environmentally benign and eco-friendly approaches for metal recovery will therefore promote a circular materials economy when one would not rely anymore from extractive - i.e., mining of the Earth materials – processes that contaminate the environment and affect human health.
We plan to address the recovery of Cobalt and Nickel from waste, which are key metals for important segments of the energy future. We plan to develop novel sponge-based reusable sorbent materials for concentrating and then releasing metal species through an eco-friendly, low temperature, hydrometallurgy approach. We believe our innovations has the potential to be applied to other metals/contaminants and contribute to broader social benefit beyond economics.
Matthew Grayson and John Torkelson
Stress Relaxation in Reprocessable Covalent Adaptable Networks
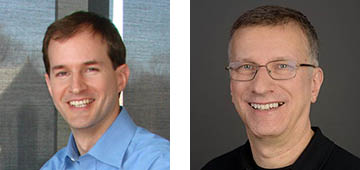
Lead-PI: Matthew Grayson. Professor, Department of Electrical and Computer Engineering
Co-PI: John Torkelson, Professor, Department of Chemical and Biological Engineering and Department of Materials Science and Engineering
Some of the strongest and most useful plastics are not recyclable because they contain permanently cross-linked covalent bonds which cannot be undone without breaking down the entire material, e.g., rubber tires. At end use, these thermoset plastics are typically burned for energy or else populate landfills or contaminate oceans. However, covalent adaptable networks (CANs), such as those developed by Prof. John Torkelson in Chemical and Biological Engineering and in Materials Science and Engineering, contain dynamic covalent bonds that can be disconnected and reconnected by heating and cooling, respectively, allowing recyclable and reusable polymers to replace single-use plastics. However, this advantage of reuse comes with a price – these CAN polymers tend to also be more susceptible to deform under stress and elevated temperatures. Fortunately, the exact way these deformations arise should hold clues to developing stronger CAN polymers. By teaming up with Prof. Matthew Grayson in Electrical and Computer Engineering, a newly developed mathematical description of relaxation processes designed to model electronic behavior in flat panel displays can be applied to the mechanical relaxation of these CAN polymers. Initial tests of Grayson’s mathematical fits to Torkelson’s data look promising and suggest that systematic studies will reveal relationships between microscopic chemical bond dynamics and macroscopic mechanical performance that had previously been hidden, leading to stronger, more environmentally friendly plastics.
Alessandro Rotta Loria and Steven D. Jacobsen
SEACRET: Strengthening Electrochemically Any Coastal Region without Environmental Threats
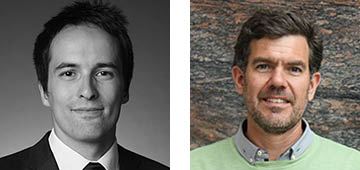
Lead-PI: Alessandro Rotta Loria, Assistant Professor, Department of Civil and Environmental Engineering
Co-PI: Steven D. Jacobsen, Professor, Department of Earth and Planetary Sciences
More than half of the world’s population lives in coastal areas and is daily affected by the detrimental effects of coastal erosion, which include coastal property loss, damage to structures, and loss of land. Currently, various approaches are available to control coastal erosion, but none of them are both effective and environmentally friendly. The research goal and intellectual merit of this project is to investigate a new, effective, and sustainable approach to strengthen marine soils and limit coastal erosion, with the ultimate aim to enhance the resilience of coastal areas against local sea level rise, strong wave action, coastal flooding, and other processes exacerbated by human-induced climate change. Specifically, this project will investigate the uncharted and potentially revolutionary path of using low-voltage electric current to strengthen marine sands via the precipitation of binding mineral crystals in the pore network of such materials. The efficacy and effects of this process, called electrodeposition, will be studied via advanced laboratory tests and theoretical analyses. By establishing a new and multidisciplinary collaboration between two research laboratories at Northwestern University, this project will develop fundamental scientific knowledge that has the potential to pave the way for a new research field at the intersection of geomechanics and electrochemistry. This research endeavor also has the promise to develop new techniques and technologies to strengthen marine soils in effective and sustainable manners. Applications of these novel techniques and technologies include the prevention of natural hazards (e.g., erosion and flooding control, slope stabilization, and liquefaction potential reduction) and the protection of marine infrastructure against coastal threats (e.g., foundation and roadbed strengthening against diffused and localized settlements).
2020 Awarded Teams
Giuseppe Buscarnera and Petia Vlahovska
Physics-based assessment of societal risks due to the collapse of mining waste disposal facilities
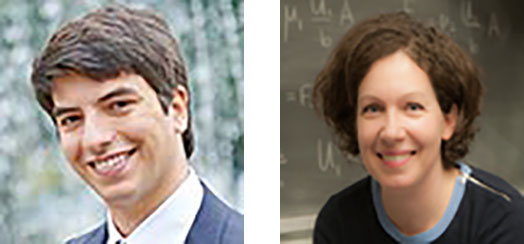
Lead-PI: Giuseppe Buscarnera, Associate Professor, Department of Civil and Environmental Engineering
Co-PI: Petia Vlahovska, Professor, Department of Engineering Sciences and Applied Mathematics
Unsustainable mining produces vast amounts of residues and the proliferation of waste disposal facilities, such as tailing dams. Due to their high toxicity and marked vulnerability to rainfall, such earthen structures constitute a major risk for the environment. Evidence from recent failures shows that tailing dams may suffer fluidization after long periods of apparent inactivity, which culminate in high velocity, long runout and loss of human life. The pervasive distribution of tailing dams around the world, combined with well-known trends of population growth and increasingly severe weather, give compelling arguments to address this problem with rigorous scientific tools. For this purpose, this project aims to formulate an innovative conceptual model bridging the pre-failure regime of tailing dams with their post-failure dynamics. By doing so, it will develop physics-based indicators of impending instability to monitor and retrofit waste disposal facilities. The outcomes of this project can therefore inspire the formulation of the next-generation of risk assessment tools to decommission facilities with intolerable hazard levels, as well as to design early warning systems increasing the resilience of communities and ecosystems to climate-induced geohazards.
Jeffrey J. Richards and Michelle Driscoll
ViSER (VIsualizing Suspension Electro-Rheology): a new tool for interrogating microstructure in fast-flowing suspensions
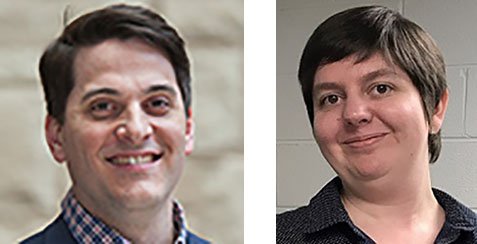
Lead-PI: Jeffrey J Richards, Assistant Professor, Department of Chemical and Biological Engineering
Co-PI: Michelle Driscoll, Assistant Professor, Department of Physics
In this seed proposal the co-PI’s, Michelle Driscoll and Jeff Richards, seek to develop a new apparatus (ViSER) to image particle-laden, high-velocity flows. Such flows are encountered in emerging electrochemical technologies and existing battery technologies that seek to reduce the cost of renewable electricity. In this seed proposal, the PI’s will focus their efforts on the development of the apparatus and obtaining experimental data from suspensions that are subjected to complex deformation that mimics realistic operating conditions. By visualizing the particles within the suspension under these conditions, the PI’s seek to bridge a gap in knowledge between predicting performance and measuring material properties in idealized laboratory settings. Successful completion of the seed proposal will pave the way to new scientific discoveries and accelerate the transition to a sustainable energy economy.
Amanda Stathopoulos and Emőke-Ágnes Horvát
Community Vulnerability Index: Examining resilience to overlapping hazards
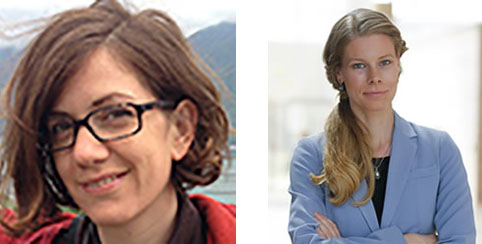
Lead-PI: Amanda Stathopoulos, William Patterson Junior Professor, Assistant Professor, Department of Civil and Environmental Engineering
Co-PI: Emőke-Ágnes Horvát, Assistant Professor in the Department of Communication Studies, (by courtesy) the Computer Science Department of the McCormick School of Engineering, and (also by courtesy) the Department of Management and Organizations of the Kellogg School of Management
It is well known that socially vulnerable populations are disproportionately impacted when disasters strike. Specifically, social vulnerability factors such as lacking social capital, or neighborhood cohesion, cause households to have less resources to deal with emergencies like tornadoes, flooding or heat waves. The COVID-19 pandemic adds a new layer of vulnerability to communities coping with hazards due to devastating health impacts and risk of contagion. Existing agency emergency management plans designed for a ‘general population’ of people who can access resources, comply with directions, and move out of harm’s way rapidly are not prepared for this new reality. Notably, disasters and emergencies occurring in tandem with the COVID-19 crisis generate new challenges for evacuation communication, transportation and logistics.
Research is needed to define community vulnerability and examine how households behave when a public health crises overlaps with acute emergencies. Our team brings together transportation engineering, social science theories and network analysis to provide a leap forward in our understanding of community resilience to simultaneous crises. We use detailed surveys coupled with crowd-sourced data to tackle three research objectives: 1) define a community resilience index accounting for social embeddedness of decision makers, 2) examine the impact of overlapping hazards, and 3) study the effect of overlapping pandemic and emergency hazards on critical decisions to comply with official guidance, timing and mode of evacuation. This proof-of-concept research promises new insight to help agencies ensure social equity in mitigation, response, and recovery from emergencies by highlighting the crucial role played by the social fabric of American communities.
Amanda Stathopoulos, lead PI on “Community Vulnerability Index: Examining resilience to overlapping hazards” has published the first paper associated with this interdisciplinary project funded by CESR:
Title: “Dueling emergencies: Flood evacuation ridesharing during the COVID-19 pandemic”
Authors: Elisa Borowski, Victor Limontitla Cedillo, Amanda Stathopoulos
Publication: Transportation Research Interdisciplinary Perspectives, Volume 10, 2021
Muzhou Wang, Julia A. Kalow, and Jeffrey J. Richards
Connecting microscopic reprocessing to macroscopic properties for a circular plastics economy
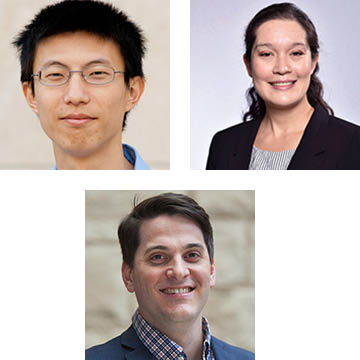
Lead-PI: Muzhou Wang, Assistant Professor, Department of Chemical and Biological Engineering
Co-PI: Julia A. Kalow, Assistant Professor, Department of Chemistry
Co-PI: Jeffrey J. Richards, Assistant Professor, Department of Chemical and Biological Engineering
Thermosetting plastics represent a $92.4B market, but since these materials are largely landfilled or downcycled at end of use, they impose a >50 megaton environmental burden. Within the past decade, many compelling solutions to the plastics pollution crisis have emerged based on materials that can be recycled by reconfiguring their chemical bonds. In principle, these dynamic bonds allow repair and reprocessing of used or damaged material, greatly extending the overall service life. However, the most fundamental steps of reprocessing remain invisible to standard experimental techniques, and the integrity of recycled materials is not tested under realistic conditions. Identifying highly recyclable elastomers and efficient reprocessing conditions requires insight that bridges disparate length scales. This project combines innovative materials design, next-generation microscopy, and advanced mechanical characterization to address this gap. For example, what engineering parameters during the recycling process determine whether the recycled and re-formed material has the same overall properties? What is happening at the molecular length scale to enable this recovery in macroscopic properties? This project will directly observe the recycling process at the unprecedented nanoscale, and correlate this to bulk mechanical properties. By visualizing the motion of individual polymers in real time, we can understand the underlying dynamic mechanisms near the interface between re-forming pieces, and how mechanical properties recover to their original state. This fundamental knowledge will help us understand which elastomers can be rendered recyclable and why, minimize the energy required to reprocess them, and maximize the retention of properties after recycling.
2019 Awarded Teams
James Hambleton and Simge Küçükyavuz
Sensing Material Properties as Nature Intends
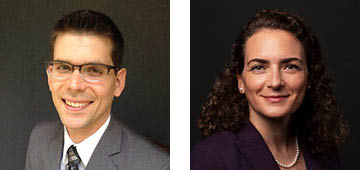
Lead-PI: James Hambleton, Assistant Professor, Department of Civil and Environmental Engineering
Co-PI: Simge Küçükyavuz, Professor, Department of Industrial Engineering and Management Sciences
This exploratory project imagines a highly non-traditional approach to devise novel test methods for characterizing the properties of natural and manufactured materials that are essential to the design of sustainable and resilient infrastructure. The central idea is that the material itself should inform the test method that most effectively reveals the material’s properties, a concept that will be explored by forging a new collaboration between researchers in Civil and Environmental Engineering (CEE) and Industrial Engineering and Management Sciences (IEMS). Existing methods for inferring the mechanical properties of materials through lab experiments or field tests have evolved largely by trial and error, and there is no general, systematic approach for evaluating one possible approach against another. Moreover, existing characterization techniques are inadequate for determining all parameters required to define the material’s behavior, particularly when the number of parameters is large. Advances in this project will enable the discovery of new devices and testing protocols that will potentially revolutionize the way material properties are measured, both in the lab and in the field.
Adilson E. Motter, Daniel M. Abrams, and Daniel E. Horton
What is the Air Quality and CO2 Impact of an Electric Vehicle Transition?
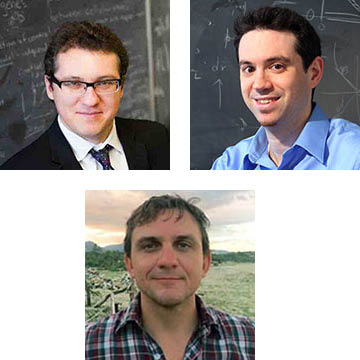
Lead-PI: Adilson E. Motter, Charles E. and Emma H. Morrison Professor, Department of Physics and Astronomy
Co-PI: Daniel M. Abrams, Professor, Department of Engineering Sciences and Applied Mathematics
Co-PI: Daniel E. Horton, Assistant Professor, Department of Earth and Planetary Sciences
This project will quantify the precise impact of the electrification of the transportation sector on the emission of greenhouse gases. Current estimates are ad hoc and rather crude due to difficulties in predicting the battery-charging power source and attendant emissions as well as the efficiency of vehicles in a post-electrification world. This pilot project will circumvent these limitations by combining multiple types of data and computational models with calculations to identify which power plants will power electric vehicles across the country. The work will use data on the actual design of the U.S. power system planned for the next several years. The expected results have the potential to inform best practices with regard to electric vehicle adoption, power generation, and greenhouse gas emission reductions.
Kyoo Chul Park and George Wells
Nature-Inspired Enhanced Microplastics Digestion-Capture and Biodegradation by Flexible Fibers with Attached Microorganisms
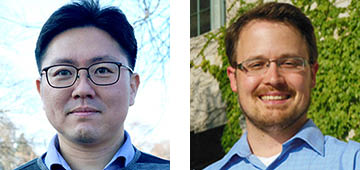
Lead-PI: Kyoo Chul Park, Assistant Professor, Department of Mechanical Engineering
Co-PI: George Wells, Associate Professor, Department of Civil and Environmental Engineering
The overarching goal of the proposed research is to engineer flexible fibers with attached
microorganisms for the energy-efficient capture and biodegradation of unwanted waterborne
microplastic particles. The proposed functional surface design approach is inspired by (i) baleen
whales that capture microscale planktons using the flexible fiber structures and (ii) mealworms that
eat plastics using the plastics-degrading microorganisms in their gut. We envision that understanding the science behind the physico-chemical and biological interaction between the microorganism enhanced flexible fibers and microplastics would lead to the development of sustainable solutions to the urgent challenge of plastic pollution.
Linsey Seitz and Niall Mangan
Multi-Scale Analysis of Electrocatalytic Reactor Processes using a Combined Experimental and Modeling Approach
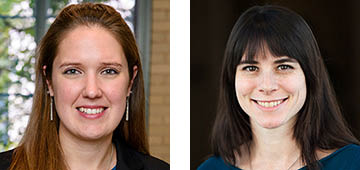
Lead-PI: Linsey Seitz, Assistant Professor, Department of Chemical and Biological Engineering
Co-PI: Niall Mangan, Assistant Professor, Department of Engineering Sciences and Applied Mathematics
Renewable energy sources, such as wind and solar, contribute a growing fraction of our global energy supply, largely due to technology advances that have improved capture efficiencies and reduced costs, even compared to fossil-based electricity. By developing sustainable processes to use the electricity from these intermittent sources to produce liquid fuels and chemical products, we can store the energy in a transportable form and buffer from their inherent intermittency. Electrocatalytic processes are a promising route to accomplish this task as they enable production of myriad fuels and chemicals, which may allow us to augment or even replace current commercial processes and significantly reduce or eliminate greenhouse gas emissions. Since electrocatalytic processes can operate at room temperature and pressure, they also open opportunities for distributed manufacturing of high-value products. Unfortunately, exciting opportunities for electrocatalytic technologies are limited by our understanding of the complex relationships between bulk reactor properties in operational devices and the local catalyst environment which influences reaction efficiency and selectivity towards desired products. Using a combination of fundamental experiments and multi-scale modeling, we will investigate the coupling between these bulk and local scales to bridge the gap between carefully controlled laboratory catalyst environments and operational electrocatalytic reactors. Our initial study will investigate electrocatalytic production of hydrogen peroxide, as a sustainable alternative to the current industrial process, which is energy intensive and produces significant waste. Hydrogen peroxide is an environmentally friendly oxidant that is used in large quantities for a variety of industries, including water treatment. Through this work, we will build fundamental understanding of electrocatalytic reactors necessary to develop technologies for effective coupling of renewable electricity from wind and solar to sustainable production of fuels and chemicals.
George Wells and Keith Tyo
Towards a Circular Bioeconomy: Recovery of Nitrogen as a Value-Added Product from Farm Animal Manure
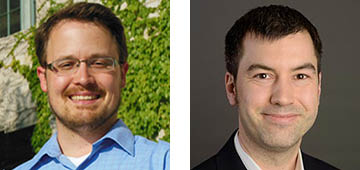
Lead-PI: George Wells, Associate Professor, Department of Civil and Environmental Engineering
Co-PI: Keith Tyo, Associate Professor, Department of Chemical and Biological Engineering
Wet wastes, including manure, municipal wastewater, and food waste, contain valuable nitrogen, carbon, and phosphorus that could be used to make fertilizers, biofuels, and bio-based chemicals in order to offset current non-sustainable production of these important goods from fossil fuels. Instead, these wastes currently require additional energy for treatment and disposal, or at worst are released, causing harm to ecological systems. We propose to develop technology to enable recovery of valuable products from wet wastes, resulting in a significant step toward a more circular bioeconomy. Support from CESR will allow us to demonstrate baseline feasibility for low-cost preparation of manure for bioprocessing and recovering waste nitrogen, a key technical challenge requiring outside the box solutions. The innovation of the proposed approach lies in recovery of waste nitrogen in a form that can be reused. The proposed work would also lay the groundwork for development of integrated technologies for recovery of carbon as a feedstock for biofuels or bioproducts, and phosphorus as a fertilizer.